The evolution of the human foot and its relation to foot and ankle dysfunction
By Lyndon Mason
Winner of 2018 Robert Jones Gold Medal and Association Prize |
During the course of human evolution the foot of our ancestors has been gradually modified in order to meet the requirements of erect posture and habitual bipedalism1. This has resulted in a structure which exhibits uniquely well-developed abilities to act as a shock absorber and a rigid lever in different aspects of gait. In the early 20th century, the concept of human evolution as the origin of orthopaedic ailments was one of intrigue and curiosity. This is especially pertinent in the arena of foot and ankle surgery, where most of the similarities of orthopaedic pathological processes and the anatomy of early hominids have been raised. Classical work by Dudley Morton in America, and Normal Lake in the UK linked the morphology of the human foot, its evolution and podiatric pathology2,3. The transformation of the human foot from an arboreal primate foot to that of bipedalism has resulted in many alterations. Firstly, a sacrifice of the grasping ability of the foot in exchange for a more lever like structure, important for propulsion. Secondly, a change in the calcaneal elevation to allow more torque conversion in the hindfoot4. Thirdly, the development of the medial longitudinal arch.
Morton’s classical work has been debated for almost a century. He proposed in 1935 a hypothetical common ancestor between humans and African apes2. Many argue that this hypothetical ancestor has now been found in the discovery of ‘Little foot’ (Australopithecus africanus)5,6. As more links in this evolutionary tree continue to be discovered a greater idea of the primordial foot is developing. Phylogenetic, ontogenetic and advancing technology are producing a plethora of new ideas and concepts regarding the aetiology and functional deficits of foot and ankle disease. In this essay, I am going to explore these ideas and functional maladies they represent.
First Ray Hypermobility
Norman Lake, published writing on the foot in 1935, where he described the development of hallux valgus as the “tendency of the great toe to return to its primitive position”3. Perera et al. systematic review on the aetiology of hallux valgus however, provided little discussion to the role of hypermobility of the first ray in its development7. Nevertheless, Morton agreed with Lake and believed that dorsal hypermobility of the first metatarsal segment was responsible for the widest array of foot deformity2. It is undeniable that the medialisation of the first metatarsal in hallux valgus deformity is a direct consequence of proximal failure or congenital deformity. Biomechanical studies are now providing evidence to Lake and Morton’s conclusions. Two papers by Kimura et al. utilising weightbearing computed tomography (CT) and a 3-dimensional (3D) analysis system to look at the differences in movement in normal compared to hallux valgus individuals, revealed a statistically significant increase in movement not only at the first tarsmetatarsal (TMT) joint but also at the other joints of the first ray in hallux valgus individuals8,9. Specifically at the TMT joint, the hallux valgus group showed significantly greater dorsiflexion, inversion, and adduction of the first metatarsal relative to the medial cuneiform9. These results are reiterated in Geng et al. paper, also noting that the first metatarsal-cuneiform joint movement can be observed in multiple planes10. Previous investigation into hypermobility as a possible aetiological cause had the significant limitations of only including sagittal motion in their definition of hypermobility, with a detailed review of the literature finding no consensus on either the direction or amount of movement of the metatarsal in normal or hallux valgus subjects11,12. Singh et al. described it as a paradox that hypermobility of the first ray was only measured in a dorsal direction, whereas a hallux valgus angle and an intermetatarsal angle are only measured in a transverse plane. These recent biomechanical studies with improved analytic technology contradicts the previous clinical papers. Wear patterns of the metatarsal head in hallux valgus specimens have also suggested a rotational movement of the first metatarsal in hallux valgus deformities, (Figure 1)13.
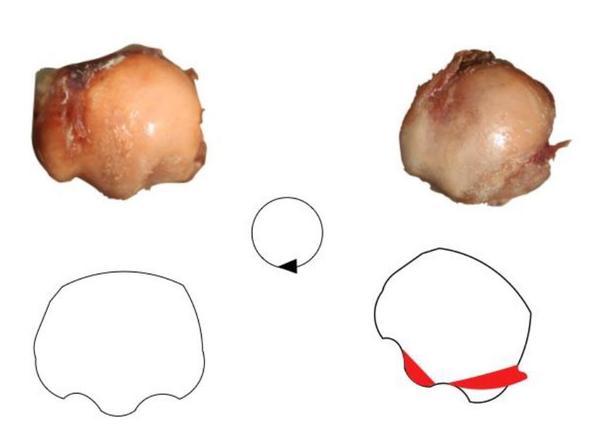
Along with the obvious mechanical differences between normal and hallux valgus individuals, the morphology of the first TMTJ has shown significant differences. Mason and Tanaka dissected 42 feet and revealed three distinct articular subtypes of the proximal articular surface of the first metatarsal (Figure 2). Type 1 had one single facet, type 2 had two distinct articular facets, and type 3 had three articular facets one of which was a lateral inferior facet connected to the peroneus longus. They found that the flatter type 1 joints occurred exclusively in the hallux valgus specimens, while the 3-facet type 3 joints occurred exclusively in normal specimens. Type 2 joints occurred in both hallux valgus and normal specimens. They concluded that the increase in facet number concurred stability. Latimer and Lovejoy dissected TMT joints in arboreal primates (Pan and Gorilla specimens) which identified very similar joint morphology to the Mason and Tanaka type 1 specimens, in keeping with the theory of an evolutionary aetiology5. Doty et al, also confirmed the variant nature of the first TMT joint14.
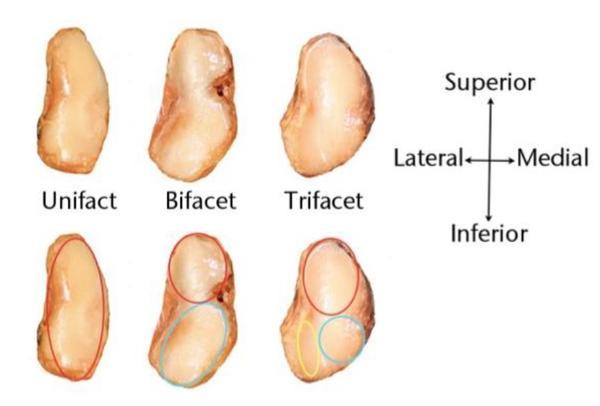
The bony and articular anatomy are not the only stabilisers of the first metatarsal. Increasing evidence has become available of the importance of peroneus longus in first ray stabilisation. In elctromyographic studies in Euprimate grasping feet, the peroneus longus muscle does not necessarily provide the grasping action of the hallux but plays a role in adducting the hallux during grasping behaviours that require stabilisation of the ankle15. Its actions in humans therefore is not too dissimilar. In a dynamic cadaveric model, Dullaert et al. illustrated the importance of peroneus longus in controlling the first metatarsal subluxation and rotation in humans, concluding that the function of peroneus longus was significantly more important in the control of the first ray than control of the medial longitudinal arch16. A follow-up study by Mason and Tanaka to their TMT joint morphology paper showed a variant nature of peroneus longus insertion with hallux valgus specimens compared to normal specimens, although they could not conclude if this was as a result or a cause of the clinical deformity17. The peroneus longus and TMTJ anatomy are crucial in preventing the first metatarsal taking up a prehensile position and thus causing hallux valgus.
Medial Arch
The medial longitudinal arch is a complex interaction between the bone shape, joint shape, acting tendons, and ligamentous supports. It acts as a shock absorber, working synergistically with these structures, including the plantar aponeurosis, absorbing, storing and transferring energy. Through the midtarsal joint, the forefoot moves upon the hindfoot to alter the action of the foot from a shock absorber to a rigid lever. For example, in the static stance phase of gait the tibialis posterior action is minimal, with the shape of the foot being predominantly dependent on the static restraints. In the propulsive phase of gait, the action of tibialis posterior supinates the midfoot on the hindfoot, causing a theorised 'midfoot lock'.
Lapidus wrote that one of the main morphologies of the human foot was its ability to absorb bending stresses so that it can be used as a functional lever18. Due to the ankle plantarflexion in the propulsive phase of gait, the medial column endures significant shearing forces. Wang et al. illustrated in modelling of a non-human ape foot in compared to a human foot in bipedal gait, a significant increase in joint force across the medial column19. One of the main adaptations of the human foot is the relative plantarflexed position of the talonavicular joint, thus preventing bending moments at this joint as the apex of the medial arch20. Any injuries that alter this joint plantar flexed position will cause dysfunction and degeneration.
In adult acquired flat foot deformity, for many years the focus has remained on tibialis posterior pathology. Specifically investigating this important tendon, Vereecke et al. described the insertion of the tibialis posterior in two arboreal primates compared to humans21. They found the insertion of the tibialis posterior tendon in the arboreal primates to be mostly the plantar side of the medial cunieform with some insertion on the navicular. The classical paper by Lewis, examined many primates and found in new world monkeys, a primitive navicular insertion of the tibialis posterior22. In old world monkeys there was a lateral plantar prolongation, reaching as far as the bases of the second, third and fourth metatarsal bones, which was elaborated and on occasion entirely replaced the navicular insertion. The author felt this may have helped with the grasping function of these early primates22.
In comparison, human feet have many tibialis posterior insertions. Multiple publications have described three discrete bands, an anterior band inserting on the navicular/ medial cunieform, posterior band inserting on the sustentaculum tali and a middle band descending to the middle/lateral cunieform, cuboid and second to fourth metatarsals23,24. Swanton et al. described an extension onto the medial cunieform from the anterior band as the navicular cunieform ligament (figure 3). This become a static restraint between two bony insertions (navicular and medial cunieform), and increases the lever arm of the posterior tibial tendon25. The increasing number of plantar insertions in humans, and the synergistic effects from connections to intrinsic foot muscles enhance the upward lifting of the arch of the foot by tibialis posterior.
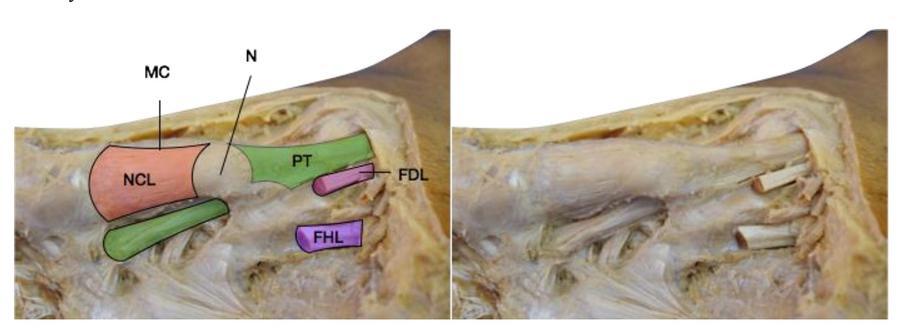
Maladaptation’s of the medial longitudinal arch can broadly be divided into high arch (pes cavus) or flat foot disorders (pes planus). The midfoot balance is essential for normal bipedal gait, and any disorder that increases or decreases the controlling muscle tension with or without normal anatomy, will ultimately lead to a pes cavus of pes planus deformity. For example, if the tibialis posterior becomes dysfunctional, the arch becomes at risk. Pes cavus on the other hand, is noted when there is an increase in tension of the controlling tendons and musculature.
Hindfoot torsion conversion
Kidd argues that as the degree of bipedality increased at the expense of quadrupedality or arboreality, there was a greater need for torque conversion at the rearfoot. In comparison to arboreal primates, humans have an elevated subtalar joint axis from the transverse plane26. Unique to humans is the enlargement of the calcaneal tuberosity and the development of the talus lateral tubercle. The calcaneal pitch, is clearly increased in comparison to the very flat primate calcaneum (figure 4). This increase in calcaneal pitch profoundly increases the subtalar torque. Morton observed that “remodelling of the calcaneum may be regarded as a key to all other important changes.”2 This is primarily due to the coincidental forming of the posterior aspect of the longitudinal arch, and its role as a motor in driving the change between the two major functions of the bipedal foot. Secondly, analysis of the gait between humans and chimpanzees show the main difference being the way in which the heel leaves the ground27. Humans have a seamless heel elevation as the foot progresses to the propulsive phase, compared to the gradual two stage heel lift in chimpanzees, combined with significant midfoot dorsiflexion.
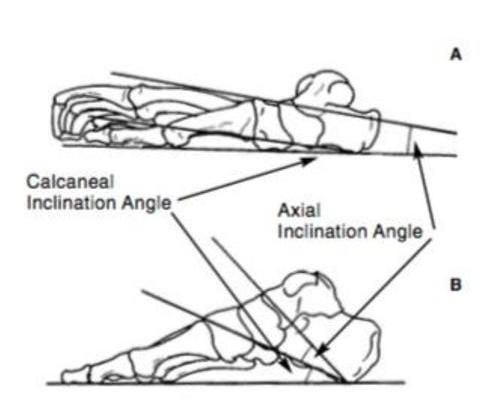
The functional maladaptions in the alteration of the hindfoot torque mechanism are multiple. Congenital deformity, such as shortening or reduced pitch, of the calcaneum will lessen the torque mechanism. Similarly, any hindfoot tarsal coalition will prevent the coupling of the subtalar and midtarsal movements, increasing the shear force and work rate in the remaining joints. In addition, any coalition or fusion of the hindfoot can subsequently drive an increase in range of movement in the midfoot, regressing to an arboreal primate gait. For example, Schuh et al. found that following double fusions of the hindfoot (subtalar and talonavicular joints), dynamic plantar pressure analysis revealed a significant increase in load in the midfoot region, and a decrease in the hindfoot plantar pressure compared to healthy controls28. Similarly, Wu et al. found in the 3D analysis that during level walking, limbs that had undergone triple fusions that there was a compensatory greater knee flexion angle during stance phase29.
Conclusion
Our knowledge of the path of evolutionary change of the foot is developing. Morton’s theories on the evolutionary origins of foot and ankle are not being disproved, but are accumulating more evidence, especially in forefoot disease. Regarding the midfoot and hindfoot, this is more complex due to the complex interactions of the changes in bony architecture, tendon insertions and ligamentous supports. When comparing humans to other primates, it is at times difficult to distinguish clearly between primitive features which are ancestral and specialised features, which are derived in their own adaptations and therefore we need to be mindful in our conclusions. Some foot pathologies are clearly 'atavistic', or reminiscent of a previously adaptive primitive condition which compromises function in the modern human foot. Others are less clear, however the knowledge of the evolutionary origins of the foot gives much better understanding in the functional normalities we possess and take for granted.
Evolution is the change in the heritable characteristics of biological populations over successive generations. This depends on the development through the natural selection of small, inherited variations, an increase in the individual's ability to compete, survive, and reproduce. The evolution of the foot therefore, had to convey some advantage. Many theories have been placed, with a change in habitat and the freeing of the hands to use tools, being the most common. It is unknown whether certain populations of humans have differing foot characteristics as a consequence of continued evolution, especially since the introduction of foot wear, but some authors do report that characteristics adapted to their surroundings are present in some30. External influences are likely to drive any further foot changes in the future. Several authors have already noted differences in shod and non-shod populations. D’Août et al. in a comparative study on barefoot Indians from the city of Bangalore and Caucasian subjects from Belgium found lower peak pressures in the unshod subjects than in their Western peers31. Similarly, Dave et al. noted a difference in deformities in rheumatoid arthritis patients32. They concluded that the external forces of shoe wear dictated the deformity, with hallux valgus being the most likely scenario in a shoe-wearing patient and hallux varus being more commonly encountered as the presenting symptom in the unshod rheumatoid foot. Further research into foot morphology in different populations will give a greater understanding to whether we are continuing to adapt to our ever-changing environments.
References
- Laitman JT. Evolution of the human foot: a multidisciplinary overview. Foot Ankle. 1983;3(6):301-304.
- Morton DJ. The Human Foot: Its Evolution, Physiology, and Functional Disorders. New York: Columbia University Press; 1935.
- Lake NC. The Foot. London: Bailliere, Tindall and Cox; 1935.
- Kidd R. Evolution of the rearfoot. A model of adaptation with evidence from the fossil record. J Am Podiatr Med Assoc. 1999;89(1):2-17.
- Latimer B, Lovejoy CO. Hallucal tarsometatarsal joint in Australopithecus afarensis. Am J Phys Anthropol. 1990;82(2):125-133.
- Clarke RJ, Tobias PV. Sterkfontein member 2 foot bones of the oldest South African hominid. Science. 1995;269(5223):521-524.
- Perera AM, Mason L, Stephens MM. The pathogenesis of hallux valgus. J Bone Joint Surg Am. 2011;93(17):1650-1661.
- Kimura T, Kubota M, Suzuki N, Hattori A, Marumo K. Comparison of Intercuneiform 1-2 Joint Mobility Between Hallux Valgus and Normal Feet Using Weightbearing Computed Tomography and 3-Dimensional Analysis. Foot Ankle Int. 2017:1071100717744174.
- Kimura T, Kubota M, Taguchi T, Suzuki N, Hattori A, Marumo K. Evaluation of First-Ray Mobility in Patients with Hallux Valgus Using Weight-Bearing CT and a 3-D Analysis System: A Comparison with Normal Feet. J Bone Joint Surg Am. 2017;99(3):247-255.
- Geng X, Wang C, Ma X, et al. Mobility of the first metatarsal-cuneiform joint in patients with and without hallux valgus: in vivo three-dimensional analysis using computerized tomography scan. J Orthop Surg Res. 2015;10:140.
- Roukis TS, Landsman AS. Hypermobility of the first ray: a critical review of the literature. J Foot Ankle Surg. 2003;42(6):377-390.
- Shibuya N, Roukis TS, Jupiter DC. Mobility of the First Ray in Patients With or Without Hallux Valgus Deformity: Systematic Review and Meta-Analysis. J Foot Ankle Surg. 2017;56(5):1070-1075.
- Mason LW, Tanaka H. The first tarsometatarsal joint and its association with hallux valgus. Bone Joint Res. 2012;1(6):99-103.
- Doty JF, Coughlin MJ, Hirose C, et al. First metatarsocuneiform joint mobility: radiographic, anatomic, and clinical characteristics of the articular surface. Foot Ankle Int. 2014;35(5):504-511.
- Kingston AK, Boyer DM, Patel BA, Larson SG, Stern JT, Jr. Hallucal grasping in Nycticebus coucang: further implications for the functional significance of a large peroneal process. J Hum Evol. 2010;58(1):33-42.
- Dullaert K, Hagen J, Klos K, et al. The influence of the Peroneus Longus muscle on the foot under axial loading: A CT evaluated dynamic cadaveric model study. Clin Biomech (Bristol, Avon). 2016;34:7-11.
- Mason L, Tanaka H. The variations in peroneus longus insertion in relation to tarsometatarsal joint type. American Orthopedic Foot and Ankle Society; 2015; Florida.
- Lapidus PW. Kinesiology and mechanical anatomy of the tarsal joints. Clin Orthop Relat Res. 1963;30:20-36.
- Wang W, Abboud RJ, Gunther MM, Crompton RH. Analysis of joint force and torque for the human and non-human ape foot during bipedal walking with implications for the evolution of the foot. J Anat. 2014;225(2):152-166.
- Elftman H, Manter J. The Evolution of the Human Foot, with Especial Reference to the Joints. J Anat. 1935;70(Pt 1):56-67.
- Vereecke EE, D'Aout K, Payne R, Aerts P. Functional analysis of the foot and ankle myology of gibbons and bonobos. J Anat. 2005;206(5):453-476.
- Lewis OJ. The Tibialis Posterior Tendon in the Primate Foot. J Anat. 1964;98:209-218.
- Bloome DM, Marymont JV, Varner KE. Variations on the insertion of the posterior tibialis tendon: a cadaveric study. Foot Ankle Int. 2003;24(10):780-783.
- Sarrafian SK. Anatomy of the foot and ankle. 2nd ed. Philadelphia: J.B. Lippincott; 1993.
- Swanton E, Fisher A, Fisher L, Molloy A, Mason L. The Plantar Support of the Navicular-Cunieform Joint. A Major Component of the Medial Longitudinal Arch. British Orthoaedic Foot and Ankle Society; 2017; Sheffield, UK.
- Hicks JH. The mechanics of the foot. I. The joints. J Anat. 1953;87(4):345-357.
- Holowka NB, O'Neill MC, Thompson NE, Demes B. Chimpanzee and human midfoot motion during bipedal walking and the evolution of the longitudinal arch of the foot. J Hum Evol. 2017;104:23-31.
- Schuh R, Salzberger F, Wanivenhaus AH, Funovics PT, Windhager R, Trnka HJ. Kinematic changes in patients with double arthrodesis of the hindfoot for realignment of planovalgus deformity. J Orthop Res. 2013;31(4):517-524.
- 29. Wu WL, Huang PJ, Lin CJ, Chen WY, Huang KF, Cheng YM. Lower extremity kinematics and kinetics during level walking and stair climbing in subjects with triple arthrodesis or subtalar fusion. Gait Posture. 2005;21(3):263-270.
- Stewart SF. Human gait and the human foot: an ethnological study of flatfoot. II. Clin Orthop Relat Res. 1970;70:124-132.
- D'Août K, Pataky TC, De Clercq D, Aerts P. The effects of habitual footwear use: foot shape and function in native barefoot walkers. Footwear Science. 2009;1(2):81-94.
- Dave MH, Mason LW, Hariharan K. Forefoot Deformity in Rheumatoid Arthritis: A Comparison of Shod and Unshod Populations. Foot Ankle Spec. 2015;8(5):378-383.